Neutrons

The neutron is one of the building blocks of atomic nuclei. More than half of the mass of matter surrounding us consists of neutrons. Due to their very special properties, free neutrons are an ideal tool, especially in the investigation of materials and basic research.
Neutrons, together with protons, make up atomic nuclei. With the exception of the hydrogen atom (H), whose core consists of only one proton, the nuclei of all elements consist of protons and neutrons. The number of neutrons is usually at least equal, but most of the time significantly greater, than the number of protons.
Some fundamental properties of the neutron:
Mass | 1,674 × 10-27 kg |
Charge | outwardly neutral |
Diameter | 1,7 × 10-15 m |
Lifetime (as free particle, stable in the atomic nucleus) | 880,1 s |
Substructure | 1 up- and 2 down-Quarks |
Nuclides and isotopes
Free neutrons interact with the particles in an atomic nucleus when they hit an atom. Their effect on the atom therefore depends on the number of neutrons and protons in the nucleus. Atomic nuclei with a certain number of protons and neutrons are called a nucleus. Nuclides with the same number of protons but different numbers of neutrons are called isotopes (of an element). While the interaction of X-rays with matter is essentially determined by the electron shell of the atoms and is therefore (almost) the same for all isotopes of an element, free neutrons interact with the elementary particles in the atomic nucleus, so that different interactions are observed for different isotopes of the same element.
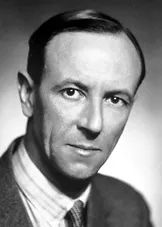
In 1932, the physicist James Chadwick conducted an experiment in which he bombarded Beryllium with alpha particles from the natural radioactive decay of Polonium. The resulting radiation showed high penetration through a lead shield, which could not be explained via the particles known at that time.
With the postulate of an uncharged (neutral) particle, of about the same weight as a proton, however, Chadwick's interpretation problems disappeared quite naturally. Thus, his results could be explained within the known laws of nature, in particular with regard to energy and momentum conservation.
Later experiments have confirmed the discovery, particularly impressive in connection with the discovery of nuclear fission by Meitner, Hahn and Strassmann at Christmas 1938.
The detection of nuclear fission and the discovery that, during the fission of heavy nuclei such as uranium, more than one neutron is released by neutron bombardment, form the basis for the generation of chain reactions and neutron beams. It took a few more years until they could be routinely used in science. The optimization process is still not finished. Machines which utilize the controlled chain reaction to produce nuclear fission are referred to as nuclear reactors.
In addition to the self-sustaining chain reaction, it is also possible to bombard suitable atoms (targets, such as lead or bismuth) with secondary particles, e.g. protons, thereby producing free neutrons. However, this reaction is not self-sustaining and comes to an end when the bombardment stops. This type of neutron source is known as a "spallation" source.
The controlled generation of intense, well-defined neutron beams requires elaborate nuclear installations, even though matter is composed predominantly of neutrons.
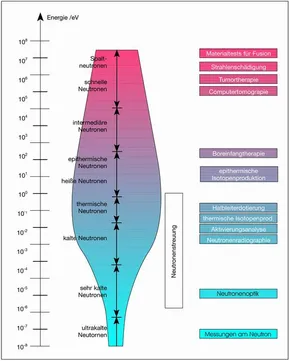
Although neutrons are a unique tool, to elicit the secrets of matter different tasks require different "types" of neutrons.
Adapt wave lengths to tasks
Neutrons as such are indistinguishable. If they are to be adapted to a specific task, it is only possible to adjust their energy and wavelength or magnetic properties. In order to define the location of atoms in a metallic compound, wave lengths of the same dimensions are necessary, i.e. 0.1 nm. To measure the globular structure of a protein, neutrons on a nanometre scale are necessary. Silicon can be very well doped for the semiconductor industry using thermal rather than fast neutrons. In the same way, in everyday life the thickness of a human hair cannot be determined using a metre stick, but is quite possible with a micrometer.
Distinguish neutrons according to their energy
It is usual to distinguish neutrons according to their energy. Depending on their energy, they are referred to as highly energetic, fission, hot, thermal, cold or ultra-cold neutrons. Moreover, it is possible through the application of suitable magnetic fields to synchronize the magnetic properties of neutrons and thus to measure the magnetic fields in the interior of solid bodies. One then speaks of polarized neutrons.
The energy of the neutrons is adjusted via moderators of different temperature. Since, in physics, the energy variables, temperature (E = kB × T), wavelength (E = ħ × ω) and speed (E = ½ mn × v2) are only different descriptions of the same phenomenon, the terms are often used interchangeably. A distinction is generally made between the following energy groups:
Description | Energy | Temperature | Wavelength | Speed |
---|---|---|---|---|
High energetic neutrons | >20 MeV | |||
Fission neutrons | 2 MeV | |||
Fast/hot neutrons | 40 – 103 meV | 2300 K | 0,05 nm | 5 km/s |
Thermal neutrons | 3 – 150 meV | 300 K | 0,2 nm | 2,2 km/s |
Cold neutrons | 0,1 – 20 meV | 25 K | 0,2 - 25 nm | 600 m/s |
Ultra cold neutrons | 10-6 – 0,01 meV | mK | 10 - 1000 nm | 5 m/s |
Equivalence of energy, temperature, wavelength and speed of neutrons. The limits are generally not well-defined. |
In order to provide neutrons with energies suitable for scientific use, the FRM II has at its disposal various so-called secondary sources. Their common feature is that they are largely located at the maximum of the thermal neutron flux, some 30 cm from the center of the moderator tank of the reactor.
Full details can be found in (pdf): Experimental facilities